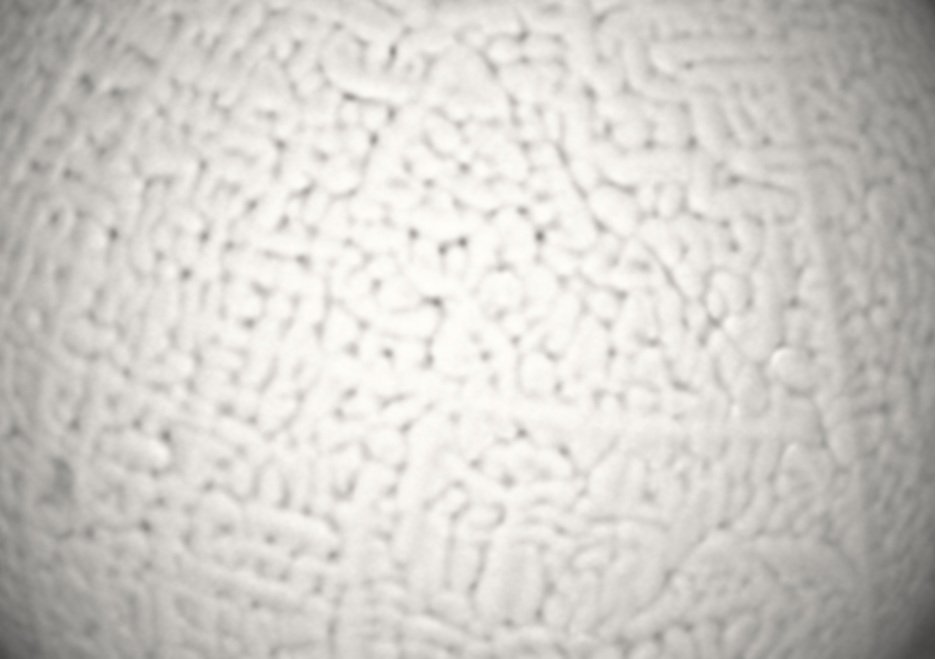
Familiarise Yourself With Common Terrestrial Spherules
During your collection journey, you may come across many different types of interesting looking grains. Some of these look very similar to cosmic spherules in colour, shape, and/or size, but do not come from space at all. Here are some common “micrometeor-wrongs” you could encounter…
But first… Can You Spot the Cosmic Spherules?
After collecting and magnetically separating dust grains, filled dishes will typically look like this. There is one cosmic spherules to look out for - can you find it? Hover over the image for the final reveal.
Anthropogenic (Man Made) Spherules
1. Road Dust
Often our samples are collected from areas close to roads such as urban rooftops or sediment exposed in road cut cliffs. Inevitably, these samples can be contaminated by particles such as road paint. You might find submillimeter-sized crystals of many different colours within your samples, and although their colours may suggest an otherworldly origin, they are in fact artificial. A closer look under the microscope should reveal a wide range of shapes and well-developed crystal faces which look angular, suggesting a terrestrial origin.
Solid glass microspheres, 300-800 microns in size, used in road and street signaling paintings. Image credit: Wikipedia
2. Industrial Spherules
Our industrial past (and present) has created a large range of spherules that can look remarkably similar to micrometeorites, especially the magnetic I-types. Most of them also contain iron, making it less straightforward to identify them by chemical analysis.
Despite this, there are subtle differences that can aid us in identifying their anthropogenic origins. Most importantly, they lack the “Christmas tree-like” cruciform dendritic or “cell-like” cellular dendritic magnetite crystals on their surfaces, and do not have an aerodynamical or perfect spherical shape. Click the accordion below for some examples.
-
Source: possibly vehicles
Appearance: dark, metallic and magnetic – at first glance, they can look exactly the same as I-type cosmic spherules
Main difference: size and morphology. Massive iron spherules usually have sizes in the millimeter-scale, which is more than 100 times larger than the average cosmic spherule. They are also usually slightly elongated, which is rare in true micrometeorites
-
Source: welding tools
Appearance: dark, metallic and magnetic – similar to I-type cosmic spherules
Main difference: morphology. Look out for spherules with strange shapes, such as composite morphologies, twin spherules, “splash” or “crack”
-
Source: mineral impurities in coal burned in steam locomotives
Appearance: metallic and possibly magnetic – similar to I-types
Main difference: colour and morphology. Spherules from steam locomotives have strange morphologies, such as composite spherules and irregularly shaped surface holes. They can also be either dark or light brown in colour, which clearly suggests that they are not micrometeorites
-
Source: construction material
Appearance: glassy and vitreous – similar to S-types
Main difference: colour, morphology and mineralogy. Mineral wool is made of melting basalt and chalk, so its mineralogy will be similar to that of terrestrial basaltic rocks – which is very different from the chemical signature of micrometeorites. They usually have tails – which is an uncommon feature in real cosmic spherules
An SEM image of a “silibead” or industrial glass bead used in many processes such as water filtering and polishing/grinding. They can come in many different sizes and are characterised by their near homogenous composition and almost perfectly smooth surface. They also appear as translucent glass in optical light. The dendritic crystals on the surface are recrystallised salt and chalk grains from contamination. Image credit: Imperial College London Micrometeorite Group
3. Urban Spherules
There is a large variety of spherules created by human activities that are present in abundance, especially within samples from an urban setting (for example, rooftops). Below is a short summary of their origins and possible appearances:
-
Source: road and roofing material
Main difference: morphology, chemistry, and surface texture.
Eroded materials from rooftops and asphalt can be magnetic or non-magnetic; the easiest way to distinguish them from micrometeorites is a simple chemical analysis. A non-chondritic spectrum suggests terrestrial origins. They also have surface textures markedly different from cosmic spherules, namely the lack of tree-like dendritic crystals on the surface.
-
Source: fireworks
Appearance: brightly coloured and plastic-like shine
Main difference: due to the addition of metal ions e.g. chromium to create different colours, some may have near chondritic chemical composition, and aerodynamical shapes, which can be confusing. The wide range of colours plus the lack of dendrites on the surface is a clear indicator of its anthropogenic origin.
-
Source: road dust and other urban activities
Appearance: glassy, vitreous lustre; non-magnetic – similar to S-types
Main difference: colour, morphology, mineralogy. These spherules come in a large range of colours; they also tend to have unique morphologies such as having multiple tails. A simple chemical analysis should also reveal silica as their major component, which does not match with a chondritic profile that micrometeorites have.
Colourful firework spherules with near perfect spherical shape and plastic-like appearance. Image credit: Jon Larsen’s “In Search for Stardust”
Natural (Terrestrial) Spherules
1. Impact Spherules
There are many spherules you are likely to encounter if you are searching through sedimentary rocks - some are just as scientifically interesting as fossil cosmic spherules with their own complex origins.
When a large extra-terrestrial body (asteroids or comets) collide with the Earth, they are travelling at more than 10x quicker than a bullet so they impart huge amounts of energy! The heat and pressure generated by the impact are so intense that they cause melting and vaporisation of both “target” (terrestrial rocks) and the impactor. As the melted and vaporised rocks cool, they form droplets known as impact spherules.
Though the formation mechanism affects their characteristics, impact spherules show broad similarities. Most are spherical, though other shapes include ovoids, teardops and dumbbells (together referred to as splash forms). Sizes are typically sub-millimetre, though can be up to 5mm in some cases.
Impact spherules are predominantly glass, though some may contain relict grains that have survived the intense heating (e.g., quartz). Some impact spherules contain small crystals known as microkrystites; those without crystalline textures are called microtektites. However, alteration of impact spherules through weathering processes may form crystals through replacement of the glass, and thus crystals may not be primary. Compositionally, impact glasses have low water content and contain traces of platinum group elements (Ru, Rh, Pd, Os, Ir, Pt), attributed to an extra-terrestrial origin.
Impact spherules may be spotted by the yellowish hue of impact glass. However, alteration of impact spherules may lead to the replacement of glass by clay minerals. The altered spherules will have a dull lustre, though mostly keep their spherical shape. Since they form in large clouds of material (called ejecta plumes), impact spherules predominantly occur in high concentrations within ejecta layers. They may also be found associated with strewn fields – areas where material is deposited from an impact event.
Yellow, glassy, spherical microtektites ~300 microns in size from the Transantarctic Mountains (TAM). Image credit: Folco et al., 2016
Backscatter electron image (BEI) of a smectite spherule. The core is full of magnesioferrite and the rim is smectite. The dendritic crystals in the interior shows this is a microkrystite rather than a microtektite. Image credit: F. T. Kyte
2. Volcanic Spherules
Volcanic eruptions produce vast amounts of dust and ash particles, many of them being thrown into the atmosphere and transported across the globe. Most appear as angular pieces of volcanic glass, but some can look remarkably similar to glassy S-types: Pele’s tears, also known as achneliths.
These are formed when magma from an erupting volcano is rapidly cooled (or quenched), resulting in small droplets of volcanic glass. They can tell us a great deal of information about the eruption such as its explosivity, composition, and even the general wind direction on the day.
A simple chemical analysis can distinguish them from cosmic spherules as they are generally rich in silicate minerals, such as Si, Al, and O which point to their terrestrial origin. Pele’s tears are also typically 2 to 64mm in size, which is ~100 times larger than most micrometeorites, and they are usually not completely black or brown, but have a mottled appearance of a range of dark colours.
A glassy volcanic spherule within volcanic tephra (particles) from the Tromen volcanic field in the Andes mountain range. Image credit: Kirstein et al., 2020
3. Organic Spherules
Small, organic material which take a spherical form can have varying interesting origins, from plant seeds to biological excrements (coprolites). If these are found in sedimentary rocks, they have become fossilised and can tell us a lot about the flora and fauna that lived in that environment millions of years ago.
Another type of common organic spherules is called carbonaceous spherules, which are dark and can be shiny like micrometeorites, but they come from wildfires. These fires don’t necessarily have to have taken place in modern times; they can be encapsulated within sediments at the time of their deposition! Due to their exceptional similarity in appearance with cosmic spherules, it is advisable to pick them up and confirm whether they are micrometeorites or not by chemical analysis. A high concentration of carbon would point to forest fire origins.
An unusually high number of carbonaceous spherules could indicate a spatially and temporally wide distribution of wildfires which could be triggered by events such as volcanic eruptions and impacts, so it is expected to find these particles along with volcanic and impact spherules too.
Photomicrograph of various seeds. Image credit: Wikipedia
4. Lightning Strike Spherules
Lightning discharges are extremely energetic weather events that occur everywhere on Earth (up to 44 strikes per second)! Lightning strikes can generate temperatures up to 1000s of degrees Celsius in an instant, which can melt and vaporise objects they strike such as sand grains, rocks, and volcanic dust. The molten and/or vaporised material may rapidly condense and solidify, forming tiny spherules. Lightning-generated phases, including spherules, are known collectively as fulgurites. Additionally, spherules formed from lightning occurring in volcanic plumes have been named “lightning-induced volcanic spherules” (LIVS).
Non-spherical fulgurites usually appear as Lichtenberg figures – fern-like, branching patterns created by electrical discharge. Lightning spherules (also known as fulgurite droplets) can share similarities with micrometeorites. Some have glassy textures and thus may look like S-type glassy micrometeorites. Others are metallic in composition and may be found embedded in the walls of branching fulgurite formations. Lightning spherules can vary greatly in size – from collections of metallic spherules <200 microns, to single occurrence droplets >1mm. Scientific research in this field is ongoing to determine the unique characteristics of lightning spherules.
BEI of an iron silicide spherule, suggested to have formed from lightning-induced fusion of sand in New Port Richey, Florida (USA). Note the dendritic lighter iron crystals and vesicles present throughout the section. Scale bar: 1mm. Image credit: Bindi, et al., 2023
5. Sedimentary Spherules and Magnetite Framboids
There are plenty of spherules of sedimentary origin that are naturally present in material recovered from sedimentary rock samples. For example, pale pink spherules of ooids - these are normally light in colour and have a pearly lustre. If they are cut open, you can see concentric rings inside caused by the near-symmetrical growth of calcite layers around a small detrital grain.
Additionally, naturally occurring metal grains, such as magnetite and pyrite framboids are found in many places. Due to their magnetism, and metallic appearance and composition, these can be easily and very commonly confused for I-types. Most, however, have well-formed crystal faces, or appear as elongated shapes with angular sides, which contrasts starkly with the sphericity of cosmic spherules. They are also slightly red in colour due to oxidation (rusting) and often times very small (<30 microns). They will not have dendritic crystals on their surface, but instead will have a framboidal morphology (small, well-formed crystals with clear edges, giving the appearance of a raspberry with angular rather than smooth bumps).
Magnetite framboid spherule, 20 microns in size, with pentagonal and hexagonal crystal faces. Note the raspberry-like surface texture, but the distinct lack of wüstite dendrites. Image credit: Imperial College London Micrometeorite Group