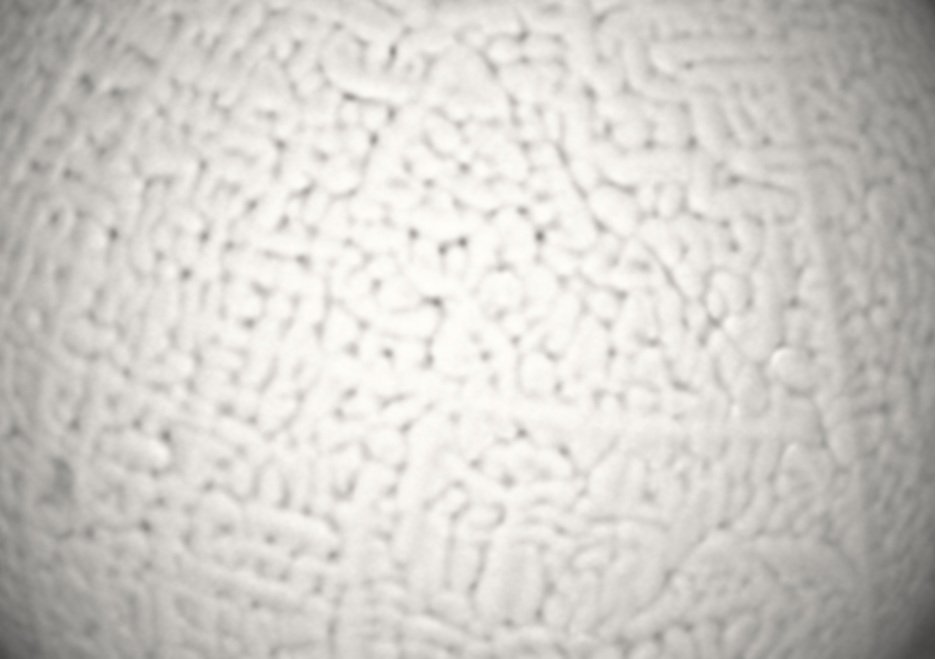
What Are Cosmic Spherules?
Cosmic spherules are micrometeorites (dust sized particles from space) that formed by melting during their atmospheric entry. They are the most abundant form of extraterrestrial material on Earth in terms of quantity and mass, and can be found all around the globe!
Scroll down to read about their history, classification, origins, sampling locations, and importance.
A brief history to their discovery
The discovery and scientific development of micrometeoritic research is relatively recent. Cosmic spherules were first described in 1876 by Sir John Murray after collecting them from deep ocean sediments during the (HMS) Challenger expedition. On this four year voyage around the globes' oceans, multiple scientific findings were made such as discovering the location of the deepest point in the Mariana Trench (thereafter named the Challenger Deep). Among many preliminary goals, one that lead to the unearthing of cosmic spherules was to determine the chemical and physical characteristics of deep sea deposits - this was done by dragging wooden planks attached to metal nets across the sea floor to disturb and catch ocean sediment and marine life. J. Murray noted the presence of magnetic spherules and later defined them as debris from falling meteorites⁽ʳᵉᶠ⁾. However, in the 1960s Donald Brownlee proved they were instead spherical cosmic dust particles due to their short isotopic ages, hence the term "cosmic spherules" was born⁽ʳᵉᶠ⁾.
Painting of the Challenger vessel by William Frederick Mitchell. Image credit: Wikipedia.
In the 20th century, micrometeorites were being found all across the Earth's surface, from beaches, to deserts, but more notably in Antarctic ice sheets. The collection and research on these Antarctic micrometeorites (AMMs) boomed from the 1980s where suddenly large quantities of relatively easily extractable and somewhat "fresh" (unaltered) spherules were now being identified.
But the presence of cosmic dust is not limited to just the surface. In fact, in 1964, Thomas Mutch hypothesised that "this same dust has been raining down on the Earth throughout geologic time" which was proven by his discovery of magnetic cosmic particles in 420 million years old salt deposits⁽ʳᵉᶠ⁾! They have since been separated from rock units of multiple ages, the oldest being an Archaean limestone roughly 2.5 billion years old⁽ʳᵉᶠ⁾!
So if extra-terrestrial particles were being found on the surface, under the surface, and below the oceans, where else could they be? Well, another way cosmic dust can be collected is with aircraft fitted with clean collector plates and sticky oils that fly at high altitudes - NASA has been accumulating these atmospheric “interplanetary dust particles” (IDPs) this way since 1981 for the Aircraft Collected Particle (ACP) Collection⁽ʳᵉᶠ⁾.
ER-2 aircraft used to collect IDPs from the Earth's atmosphere for the Aircraft Collected Particle (ACP) collection. Image credit: Nasa
The advancement of scientific equipment and sampling techniques throughout the years has lead to an increase in micrometeoritic research. However, scientists still had critical questions such as:
Where is most of the cosmic dust found on Earth coming from? Comets? Asteroids?
Why do they all have such unique and interesting shapes?
How do we know they are certainly from space?
To help find answers, two vital and well cited papers were created which helped the community identify and classify many different types of micrometeorites, and better understand the changes they go through when they enter the atmosphere. And now sampling was about to to get even easier…
Speculations that modern-day extraterrestrial dust can be acquired in urban environments began to arose as early as the 1940s, but a definitive collection emerged just recently in 2015 by Jon Larson, a private cosmic spherule collector and Norwegian musician, as part of “Project Stardust”. Now spherule collecting is no longer limited to scientists with expensive equipment - as the knowledge of micrometeorites’ significance and abundance spread, more and more of the public began finding their own samples.
Maybe you can be one of them ;)
Jon Larson collecting dust from a rooftop in Oslo, Norway. Image credit: Jon Larson and Sciencefriday
What do they look like?
Cosmic spherules are classically described as being small, dark, shiny, and spherical. They are defined to be less than 2mm in size, but their size distribution peaks at 200 microns (0.2mm). Their shape and colour also vary- they are mostly black but brown, grey, and dark red varieties also exist, and they can be ovoidal or slightly irregular in shape. Cosmic spherules acquired from the deep sea and sedimentary rocks are often altered by chemical weathering experienced throughout the years, causing some to lose their smooth shiny surface (slight speckles may still be seen though).
The identification of micrometeorites closely follows the classification scheme developed by Genge, et al., 2008, which describes not only their optical properties (i.e., what they look like under a normal light microscope), but also their fine detail textural and chemical attributes (analysed using a scanning electron microscope (SEM)). There are three main groups of micrometeorites (MMs):
Melted MMs (cosmic spherules)
Partially melted MMs (scoriaceous), and
Unmelted MMs (many varieties).
A collection of urban rooftop cosmic spherules. These are the most pristine S-types collectors can encounter due to their limited time spent on the Earth surface before imagine. Image credit: Project Stardust
Cosmic spherules (CSs) are the most abundant and show considerable diversity in textures, compositions, and mineralogy so can be subdivided into several groups, the main being:
The iron-rich variety (I-type)
The intermediate type (G-type), and
The silicate-types (S-type).
It is very difficult to determine the type of spherule you have using a low magnification optical light microscope, but a classic distinction is the size: if the sample is bigger than 0.1mm, it is very likely an S-type. Under higher magnifications, some surface patterns and variations in colour may be observed, for example: if a spherule appears glassy and almost translucent, it’s probably an S-type, but if it is dark with lighter grey dendrites (crystals in a tree-like pattern) it could be an I-type.
The S-types are further divided into multiple sub-types based on appearance and composition which can be better identified using high power electron microscopes found in research laboratories. Their detailed descriptions can be found in the gallery page.
Example images of different S-type cosmic spherule textural subtypes, presented as scanning electron (SEM) and optical images. Notice that not all are perfectly spherical, and some have slight variations in colour. Image credit: Suttle et al., 2021
A common question made by first time collectors is “what type of micrometeorite am I most likely to find?” This depends on where you are collecting your samples and how long they have been exposed to terrestrial weathering. Weathering is the deterioration of materials through contact with water, the atmosphere, or organisms. The intensity of this deterioration depends on the contact medium (e.g. salt water in the deep sea or fresh in the Antarctic), and particles that are most susceptible are made of more “fragile” (reactive) elements - in this case, the S-types. Therefore, on urban rooftops where samples have fallen in the last few years, S-types are the most abundant at >97%⁽ʳᵉᶠ⁾! This percentage drops slightly to ~95% in Antarctic collections and deep-sea sediments⁽ʳᵉᶠ⁾. In fact, the only place where I-types dominate is in ancient sedimentary rocks where S-types become significantly rare (<2%)⁽ʳᵉᶠ⁾. Finally, G-types in all environments are extremely uncommon (<1%)⁽ʳᵉᶠ⁾.
How do they form and where do they come from?
The origin of micrometeorites on Earth have been under much debate in scientific literature. The majority of cosmic particles are generated during collisions between asteroids, and ablation of dust and gases from comets as they enter the inner solar system. The distribution of the spherule abundances between these two categories, however, is not agreed upon.
It’s extremely difficult to link cosmic spherules to parent bodies because their textures and chemistry are altered when they melt during atmospheric entry. However, they are thought to be largely samples of primitive solar system objects, likely derived from asteroids with affinities to CI, CM and CR chondrites* due to their similar compositions⁽ʳᵉᶠ⁾.
*These are carbon-rich stony meteorites that have not been modified by large-scale melting whilst on their parent body and thus represent the most unaltered building blocks of planets and asteroids that were present during the start of the solar system.
A slice of Allende, a carbonaceous meteorite showing circular chondrules - a rounded grain formed as molten or partially molten droplets in space before being accreted to their parent asteroids. Image credit: Wikipedia.
One way in determining the source of CSs comes from oxygen-isotope studies. Oxygen has three isotopes that behave slightly differently to each other: 16O, 17O, and 18O. On Earth and the Moon, these isotopes are distributed in the same proportions, but on all other objects in the solar system, the proportions are different. If we can measure the precise isotopic composition of CSs, we can match them to meteorite classes with known distributions.
This is not as easy as it sounds - as micrometeorites undergo atmospheric heating, they lose more of the lighter oxygen (16O) through evaporation and react with the oxygen in our atmosphere, causing their initial isotopic proportions to change. With some assumptions on how this process works, scientists can back-track what they think the initial oxygen isotope composition of a CS is to better understand their origin.
Meteoroids burning up as they enter Earth’s atmosphere causes them to interact with atmospheric oxygen, changing their initial oxygen isotopic ratios. Image credit: Britannica.
I-types likely come from metal grains within ordinary chondrites (a primitive meteorite which is the most common on Earth), but some could also originate from bodies composed almost entirely of iron. Since I-types fully oxidise during entry, all of their oxygen is derived from the atmosphere, so researchers measure their ratios of nickel and cobalt instead of O-isotopes⁽ʳᵉᶠ⁾.
This also has its problems because of two major issues:
Not all bodies in space have a unique composition for their metal grains.
During oxidation of I-types, an inner metal bead is created of non-oxidised iron. When the outer region and inner bead is molten, elements can move between the two, and nickel, for example, preferentially goes inwards to the bead. In some cases this bead is ejected whilst in the atmosphere, taking most of the nickel with it. This ultimately changes the “left over” composition and element ratios of the I-type.
Back scattered electron images (BEIs) of metal bead bearing I-type cosmic spherules from Antarctica. The beads are not oxidised during entry through the atmosphere, but can be weathered during their time on Earth’s surface. Image credit: ICL MM research group.
-
To help us better understand where cosmic dust comes from, it is also important to grasp the differences between comets and asteroids. An asteroid is a rocky, metallic, or icy body that orbits within the inner solar system that is too small to be considered a planet. They can be various shapes and sizes (from 1m to 1000km) and can be grouped together based on their orbit locations (named “families”) or their spectral type. The spectral type of an asteroid is measured using astronomical spectroscopic instruments which measure the spectrum of electromagnetic radiation emitted by various bodies in space. The detected wavelengths of the radiation depends on the composition of the emitter, for example, “C-type” asteroids are spectrally dark in colour, meaning they reflect little optical light - this is likely because they are carbon-rich.
Comets can be considered as “dirty cosmic snowballs” as they are mostly composed of frozen gases and rock dust. They typically have very eccentric orbits and when they approach closer to the sun, their body warms and the gases and dust are released as tails in a process called “outgassing”. Comets are split into groups called “dynamic families” based on their orbital characteristics. They originate from the outer colder regions of our solar system, hence their high amounts of ice.
So the main difference between the two groups is: asteroids are mostly rocky with bits of metal, and comets are a mixture of rock and ice. This distinction arises due to where in the solar system they formed around 4.5 billion years ago. The further a body is formed away from the sun, the more likely it is to contain volatile components (chemical elements and compounds that can be readily vaporized) that are otherwise blown away by the hot inner temperatures of the solar system. The “snow line” or “frost line” is a general orbital distance from the sun where water-ice (and other ices) can condense - for water, this is around 5AU (roughly the distance between Jupiter and the Sun). This explains why the inner planets and asteroids are rocky and the outer planets and comets are made of gas compounds. However, during the formation of the solar system, this dynamic was not as stable as now. The early solar system was a disorderly place where rocky bodies were continuously colliding with each other causing some planets to grow and others to break apart. Throughout this chaotic growth era, orbits of multiple bodies frequently changed as planets’ gravities interacted with each other. This meant that bodies composed mostly of rock and metal (what we would now consider as “asteroids”) were being flung past the snow line where they could accrete high amounts of ice and dust, making them appear as comets. These comets could have very similar compositions to asteroids, which adds to the confusion of where most of our micrometeorites truly come from…
Where can we find them?
The main sampling locations for cosmic spherules briefly described above can be split into the following:
The Antarctic
Deep-sea sediments
High altitude atmosphere
Ancient sedimentary rocks, and
Urban rooftops
Each location has their own unique differences in collection methods, recovery abundances, and average appearance of spherules. Although these categories are relatively broad, the datasets they provide can be divided further based on things such as specific sampling locations, the organisations that sponsor the search, the type of general sediment recovered, etc. Explore our collection methods and datasets sections to find out more.
The first three areas are sampled by scientists who are able to organise searching expeditions that are supported by grants - these are thus difficult collections for the public to contribute towards. However, sedimentary rocks and rooftops are often easily accessible and involve little to no specialist equipment to search through. Thus, more and more of the public are getting involved in finding their own micrometeorites! If you want to join them, check out collection methods for more info.
Geologists collecting rock samples from the K-Pg boundary (the layer representing the time the dinosaurs died, 66 million years ago) in a quarry in Stevns Klint, Denmark. Image credit: ICL MM research group.
Why are they important?
Besides from being pretty to look at and fun to hunt for, cosmic spherules play a vital role in helping scientists better understand our planet and solar system processes. Although their small size limits the information that individual particles provide, their high quantity and diversity provide valuable insight into dust-forming bodies in the solar system, particularly those not sampled by meteorites.
Micrometeorites have been shown to be rich in essential life-forming elements such as carbon and phosphorous (an otherwise limiting element), and organic molecules including amino acids! All organisms on Earth contain these components in their cells, so perhaps our origins venture far out into space and were delivered by tiny cosmic particles. This links to a theory of abiogenesis (natural life-forming processes originating from non-living matter) called panspermia, meaning “seed of life”. Panspermia hypothesises that the pre-biotic organic building-blocks of life originated in space and once they landed in a flourishing environment with habitable parameters such as liquid water, these primordial ingredients generated living organisms.
-
Cosmic dust is the most abundant type of extraterrestrial material on Earth. Their flux has been estimated to be >1000 tonnes per year compared to less than 10 tonnes for bigger bodies such as meteorites⁽ʳᵉᶠ⁾. Also, their small size plays a vital role in their catalytic nature because smaller particles have higher surface area to volume ratio, and most chemical reactions takes place on the surfaces of objects.
-
Cosmic spherules commonly contain iron metal beads in their interior which is closely associated with magnetite rims/shells present on the surface. It has been proposed that these magnetite “shields” provide internal organic compounds protection against: high heat during atmospheric entry, and; aqueous dilution of chemical compounds upon deposition in oceans⁽ʳᵉᶠ⁾.
Also, these metal beads often weather to form a hydrated mineral called ferrihydrite which portrays important characteristics as a catalyst/host phase of complex organics. Ferrihydrite contains abundant nanotubules, which increase porosity and surface area to volume ratios, and it has “point defects” in its crystal structure, making it a good absorbent for phosphate and organic materials. It is also present in the core of the universal intracellular protein ferratin, meaning it may have been an important compound associated with the formation of life in early Earth⁽ʳᵉᶠ⁾.
Spherules can also contain ferrihydrite “nuggets” enriched in C, P, O, and S, meaning they could also be a protective host phase of amino acids during entry which increases their likelihood of survival⁽ʳᵉᶠ⁾.
Ultimately, spherules with a protective shell, catalyst metal bead, and high organic components have all the ingredients necessary to form a primordial life soup, especially if it was deposited in an ocean with warm liquid water.
-
Yes! Cosmic dust exists throughout the universe, even between solar systems! This means they can fall on any planet or asteroid surface including our close neighbouring planets. In fact, the high abundance of dust on the surface of Mars poses a problem when landing space missions try to measure the concentration and types of organics present on Mars because the detected signals may come from recently fallen micrometeorites rather than potential organisms that live long ago.
However, it is important to consider the atmospheric conditions of planets to determine if the organic compounds delivered with cosmic spherules can survive atmospheric entry…
-
Atmospheric heating is an important process which significantly alters the texture and chemistry of cosmic dust, but how does it occur?
Imagine a feather dropping down to the ground. Now compare this to a spacecraft re-entering the Earth's atmosphere after it's journey in space. You may picture the feather as slowly and softly falling, whilst the spacecraft is covered in flames as it quickly descends (before a parachute is diploid). Both the feather and spacecraft encounter gas particles during their fall, but the faster an object falls through the atmosphere, the more gas particles it will rub against. In fact, re-entering spacecraft travel at around 7km/s⁽ʳᵉᶠ⁾, whilst cosmic dust can enter the atmosphere at speeds of up to 72km/s⁽ʳᵉᶠ⁾! This causes a massive amount of frictional heating which can ultimate melt the particle entirely at temperatures around 1200-1500ᵒC⁽ʳᵉᶠ ¹⁾⁽ʳᵉᶠ ²⁾.
So if cosmic dust can reach temperatures which ultimately melt silicate crystals and even iron, how could organic compounds with much low melting points possibly survive? Well, not all cosmic dust encounters such high temperatures. The peak temperature experienced by particles entering the atmosphere depends on multiple factors such as it's initial size, entry velocity, and entry angle. Particles entering at high velocities and entry angles are likely to be from comets and, if these are large enough, they can evaporate entirely during their journey through the atmosphere. However, cosmic dust travelling at lower speeds of 12km/s which are small (less than 50 microns in size, or less than the width of a single human hair) will fall to the Earth's surface completely unmelted⁽ʳᵉᶠ⁾.
Unmelted or partially melted cosmic dust are therefore the most likely to contain organic compounds. Cosmic spherules, being fully molten, may have organics with altered chemistries since their volatiles (less-dense gaseous elements) easily vaporise. Some spherules, however, may have interiors which have experienced much lower peak temperatures than its surface and can contain completely unmelted minerals (called relict grains) and relatively unchanged organics which have survived entry!
Water is an important component in forming life, but is there any water in space? In meteorites and micrometeorites, the most direct evidence of aqueous alteration (changes caused by the presence of water) is the presence of clay minerals (phyllosilicates). This figure shows backscattered electron images (BEIs) of secondary mineral phases in carbonaceous meteorites grown due to the presence of water on its parent body asteroid. (a) fibrous grey serpentine (a hydrated mineral), (b) tochinilite (white) and serpentine (grey) (hydrated minerals), (c) framboidal magnetite (an oxide mineral), and (d) calcium-carbonate with twinning textures (cross-cutting lines). Image credit: Chan and Zolensky, 2022.
As cosmic dust rains on Earth, it falls through our atmosphere, undergoes heating, and reacts with atmospheric gases. Since cosmic spherules have been falling on the surface throughout Earth’s history, they have been passively sampling and tracking the change in our atmosphere’s composition. Spherules form at altitudes above 80 km, where frictional heating of small meteoroids encountering gas particles causes them to flash melt at temperatures above 1,500 °C⁽ʳᵉᶠ⁾. Spherules interact strongly with the atmosphere, incorporating terrestrial oxygen when they are molten - this causes I-types to oxidise into wustite and magnetite, leaving behind a smaller inner metal bead of non-oxidised iron-nickel.
The Earth’s oxygen levels were thought to be much lower than present a few billion years ago during a geological period called the Archaean. However, spherules acquired from Archaean limestone show that the upper atmosphere was just as oxygenated as it is today! Evidence of low oxygen levels at the surface may imply that there was minimal mixing between the lower and upper atmosphere during this time⁽ʳᵉᶠ⁾.
The upper atmosphere of Earth.
The abundance of micrometeorites on the Earth’s surface reflects the extraterrestrial dust flux being accreted to the Earth and the size distribution of particles within the Zodiacal cloud (the interplanetary dust cloud in space which is responsible for the zodiacal light). Roughly 1,600 tons of cosmic dust fall on Earth every year - that’s ~100x more than the mass of fallen meteorites⁽ʳᵉᶠ⁾! The different types of micrometeorites formed due to heating during atmospheric entry also relates to the entry velocity of dust particles, and certain textures can even be used to better understand the behaviour of dust in space prior to their entry. All of this helps us in determining key solar system events such as nearby asteroid collisions and cometary flybys, but how?
We can reconstruct the flux of extraterrestrial dust to Earth in the past by estimating the abundance of fossil micrometeorites present in older sedimentary rocks. By identifying the average fossil micrometeorite size, type, and common textures found in a rock unit, we can assume where the majority of this dust came from in space. For example, smaller micrometeorites are unlikely to survive atmospheric heating unless they have a high entry velocity - this is usually the case for particles coming from bodies with eccentric orbits such as comets! Enrichments in past dust fluxes have been used to support the discovery of the Ordovician break-up event - an asteroid collision occurring 467 million years ago which is responsible for most of the L chondrites (the second most common meteorite) being delivered to Earth today⁽ʳᵉᶠ⁾!
SEM image of an I-type spherule recovered from the Precordillera and the Zenta Range in Cordillera Oriental (Argentina). Note the extremely large escape texture, possibly from an ejected metal bead. Image credit: Voldman et al., 2012.